TISSUE ENGINEERING
from petri dish to patient
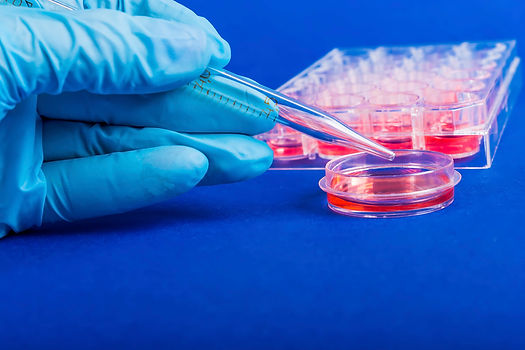
TISSUE ENGINEERED ORGANS
A MAJOR APPLICATION OF TISSUE ENGINEERING
is the ability to create various organs of the human body.
With the combination of certain cells, scaffolds, and other biomaterials that correctly match in vivo conditions of the corresponding organ, almost any organ could be made. But it is easier said than done.
With tissue engineering’s (TE) potential to create new organs, doctors may have a new method to cure or restore the function of degenerated tissues which ultimately compromise organ function and human life. Current therapies which aim to fix these problems include transplants, drugs and mechanical devices. Although these methods have shown promise, they are still plagued with limitations. These issues with current therapies may be solved with further development and increased research on TE organs.
Read more below on a selection of a few organs and TE's far-reaching impacts on disease treatments and human life.
CLICK TO READ MORE
PANCREAS
The pancreas is an organ that consists of two parts: the endocrine and exocrine. The exocrine cells produce enzymes which aids food digestion and the endocrine is responsible for releasing hormones like insulin and glucagon into the bloodstream to regulate blood glucose levels. The cells that carry out insulin production are known as islets of Langerhans. Diabetes is a disease in which pancreases either fail to produce insulin (Type 2) or the immune system destroys the β-cells of the islets (Type 1).
Current therapies include periodic insulin injection and islet and pancreas transplants. These therapies are widespread but not completely viable because of negative side effects: injection may cause hypoglycemic attacks and transplantation may cause rejection and patients have to use immunosuppressants. Another limitation is that there are not enough organs for transplantation, and if there were, there would still be risk for immunological rejection. Even islets that are transplanted are rejected by the human body. The lack of positive therapies will soon change when scientists will be able to develop fully grown pancreases.
Challenges scientists must solve in the future include finding a combination of materials that does not require immunosuppressants and acquiring more and extending the lifespan of transplanted islet cells.
Research shows positive results of using Matrigelâ„¢ matrices and fibrin scaffolds to engineer pancreases. Also, autologous stem cells are potential cell sources which is the best option for cell choice because its does not rouse ethical controversy and that there is no risk for rejection.

Anatomy of a pancreas (I7.9)
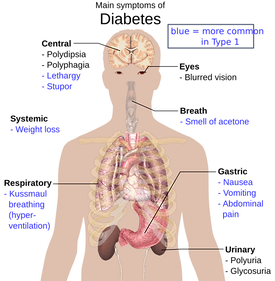
Complications of diabetes that TE pancreases hope to fix (I7.10)
KIDNEYS
The kidney is an essential organ that filters out our bloodstream and excretes the waste products in our urine. Without kidneys, waste products and liquids would build up in our system, electrolyte levels would be unstable, and we would have a lack of hormones vital to blood and bones.
Current research finds kidney construction a huge challenge due to the convolutedness of the specific arrangement of cells that compose a kidney. In the creation of engineered kidneys, scientists use a mixture of stem cells and expect them to differentiate and develop like a kidney would in a developing embryo.
With tissue engineering, kidneys, like many organs out there, can be constructed in vitro and transplanted into a patient. There high hopes for engineered kidneys in the future because surgeons can carry out renal transplants without having to go through the time-consuming process of dialysis. Like the drug therapies for heart diseases, dialysis does not necessarily guarantee protection from further degradation of the tissue. Furthermore, patients will not have to suffer from the complications that arise with the use of immunosuppressants because constructs can now be made from autologous cells. Before, kidneys were xenotransplanted from porcine which comes with a risk of immunological rejection and other diseases.
Scientists who are developing in vitro kidneys face more challenges in the growth of larger, transplantable kidneys, inability to use adult induced pluripotent stem cells, and incomplete development which lacks certain essential parts for organ function, like the ureter.
Kidney transplants are the most common organ transplants in the United States. As a result, patients who have gone through dialysis treatments now wait for a organ donor, which on average takes about 4 years. Like patients with heart diseases, those who have end stage kidney disease must eventually find an organ transplant due to continual deterioration of the tissues and the failure of present therapies to reverse tissue damage. Current transplantation requires the patient to take immunosuppressants to reduce the risk of rejection. With these drugs also come unpleasent side effects which often make the patient suffer even more.
HEARTS
The heart is a vital organ of the human body that is responsible for pumping blood through the veins and arteries to supply nutrients and oxygen throughout our body. Currently, doctors use drug therapies for patients with heart disease, however, drug therapies do not restore the heart to a healthy functioning state, but stem the inevitable degeneration of the entire organ system. Ultimately, the patient will need a heart transplant which is another challenge that patients and doctors face. Heart transplants inavailable due to the dearth of organs and the risk of organ death during transportation.
Scientists currently are focusing on construting the myocardium, the section of the organ that is responsible for contraction.
Research finds show that embryonic and adult stem cells are the most optimal cell sources for engineered organs. However, embryonic stem cells may induce tumor growth or cause heart palpitations.
Another challenge other than finding a good cell source is finding a scaffold that not only promotes cell proliferation but also mimics the bioactivity and interations of the native tissues. Scientists need to incorporate conductive materials into the scaffold to induce contractions. Current research on scaffold materials like poly(lactic-co-glycolic acid) (PLGA) and a poly(1,8-octanediol-co-citric acid) and poly(L-lactide-co-caprolactone) composite showed higher cell retention and tensile strength compared to other synthetic materials. Natural materials like collagen and chitosan also demonstrate potential viability in construts, however, collagen doesnt have the mechanical strength to support a fully grown organ. Chitosan is very versatile in its ability to be modified to exhibit favorable characteristis, such as mechanical strength or cell differentiation.
Current research on tissue engineered hearts seek to replace drug therapies by constructing personalized and functional hearts for patients with damaged tissues or heart disease. Scientists have injected stem cells into the patient’s myocardium, the part of the heart responsible for contracting, as a temporary treatment. Usually, the differentiated stem cells die off and the patient still suffers.
Engineering hearts is not impossible: Doris Taylor, the director of regenerative medicine at the Texas Heart Institute created a beating rat heart in 2008.
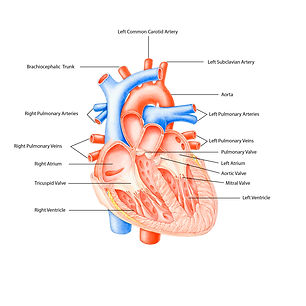
Anatomy of a heart (I7.2)
Cross section of heart wall (I7.11)
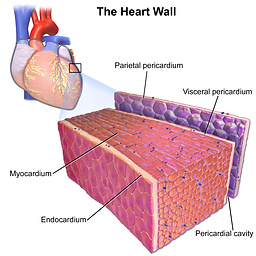
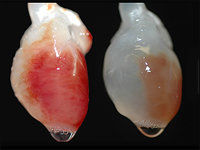
Taylor's lab grown rat hearts (I7.3)
VASCULATURE
Vascularization is the formation of blood vessels and capillaries in living tissues and is essential to creating healthy, functional organs to be transplanted in vivo. Look @ anatomy in the image at the right. Blood vessels are very important in distributing oxygen and nutrients and retrieving waste products from cells.
Researchers are creating new scaffold designs to induce and promote vascularization. Scaffolds are vital in vascularization because the rate of the process is determined by its design. Important variables include pore size and interconnection of the scaffold. Researchers are also incorporating growth factors to induce angiogenesis.
In vitro and in vivo prevascularization is another method of vascularizing tissue engineered constructs. In vitro pre-vascularization does not require a second surgical operation that in vivo pre-vascularization does. In vivo pre-vascularization is commonly used in skin, muscle, and bone tissue regeneration. A drawback of in vivo pre-vascularization is that the cultured endothelial cells do not have the ability to regulate vessel development and anastomosis, or connection between blood vessels. On the other hand, in vivo pre-vascularization generates fully-developed and organized vasculature. As stated earlier, the in vivo method requires two surgeries: first, to ensure appropriate vascularization by connecting the graft to an irrigation system, and the second one to implant the whole graft into the patient.
Vascularization is separated into two parts: vasculogenesis and angiogenesis. Vasculogenesis is formation of the first blood vessels and angiogenesis is the formation of new blood vessels from present ones in response to growth factors or signals from the ECM.
Researchers are currently researching vascularization in tissues to further comprehend diseases like cancer and the vascularization of engineered tissues (a major challenge in TE organs atm). Vascularization is essential in TE because it mimics in vivo conditions in that there is a transfer of waste products and oxygen throughout the construct and boosts natural regeneration of damaged tissues.​

Vasculature throughout the human body (I7.1)
TRACHEAS
The trachea, also known as the windpipe, is a hollow organ which is acts as a channel for respiration. Diseases like cancer threaten the functionality of the trachea, ultimately putting the patient’s life on the line.
Although possible, engineered tracheas face a long road until they are widely used as treatment options as scientists need to further research on optimal biomaterials and organ growth.
Current tissue engineered traheal development faces challenges from the complexity of the trachea itself, consensus on the cell source, optimal materials for scaffolds that the body does not reject, proper characteristics and vascularization throughout the organ, and finally, organ growth in vivo.
Current therapies are limited to resection and anastomosis (cut and sew), suboptimal laser treatments, and stents. Many problems are associated with these techniques which include inflammation, dissimilarities between native and transplanted allogenic tissues, incomplete integration, and collapse. Engineered tracheas have not been developed enough for clinical applications.
Despite challenges, trachea transplants are not impossible: Paolo Macchiarini completed several engineered trachea transplants. He first operated on a 36-year old male with a trachea made of stem cells and a scaffold made of nanocomposites. He also injected autologous epithelial cell into a trachea scaffold decellularized by enzymes and transplanted the trachea into a 30-year old female. Read more about Macchiarini's achievements here.
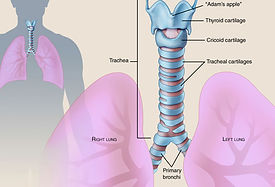
Trachea connection to lungs (I7.8)
BLADDERS
The bladder is an organ that holds urine for excretion and can be compromised as a side effect of a wide variety of injuries and diseases such as cancer, trauma, infection, or inflammation.
Patients who have bladder problems must go through bladder reconstruction, also known as augmentation ileocystoplasty. Cystoplasty is very risky because it reconstructs the damaged tissue in the bladder with gastrointestinal tissue. Gastrointestinal tissue absorbs solutes the bladder intends to excrete. It also causes an electrolyte imbalance, infection, metabolic disruption, and much more unnecessary and enervating complications.
Engineered bladders are currently created from differentiated stem cells which prevents complications of the bladders made of gastrointestinal tissue. Bladder constructs are relatively affordable and easy to make, due to its hollow structure and simplistic mechanism. Anthony Atala has completed 7 successful bladder transplantations; these transplanted bladders fully developed safely in vivo 5 years after the operation.

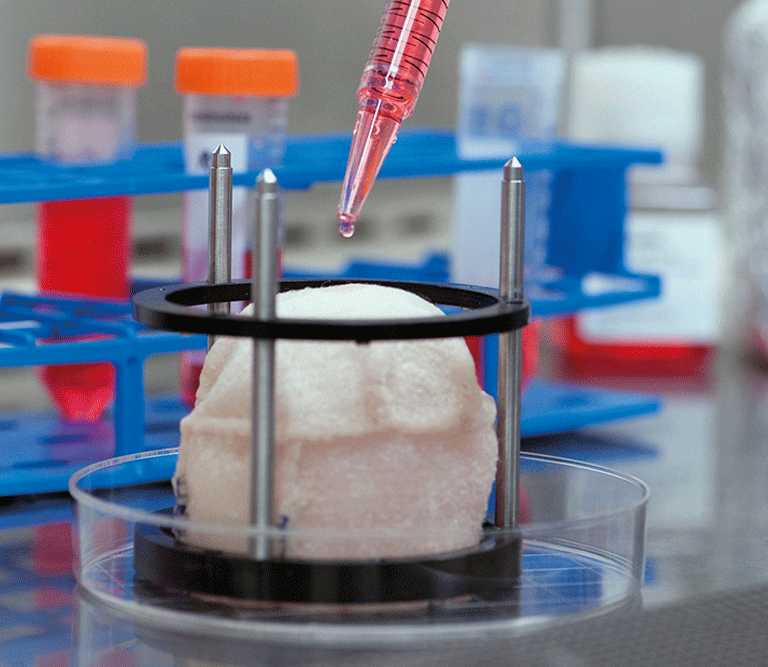
Anatomy of a bladder (I7.6)
In vitro bladder construct (I7.7)
BONES
The skeletal system consists of calcified tissues known as bones. Bones are natural scaffolds which protect vital organs and support the body. These organs are formed by two layers of tissue: the outer layer, which is not very porous, but provides the organ with its high mechanical strength, and the porous inner layer with reduced mechanical properties. Because of its features, bones are challenging to construct in vitro, but demonstrate a great potential in medical applications.
Unlike the aforementioned organs, bones have a greater capacity to regenerate and repair itself when damaged, but only to a certain extent. When bones reach their reparative limit, especially in situations when bone damage is too complex or severe, the bone cannot repair damage. Extensive bone damage requires a patient to receive a bone graft transplantation to help restimulate restorative growth.
Currently, bone grafts are created from autologous cells harvested commonly from the iliac crest. Autologous bone grafts are standard treatments, but limited, because there is a sparse amount of cells of this type. Alternatives include the usage of allograft, a graft from another human, or xenografts, a graft from another species. These alternative alternatives are unpopular because of possible risk of infection, disease, and immunological rejection.
Scientists are constructing bones by using porous scaffolds that are similar to the composition of the bone. The bioceramic, hydroxyapatite (HA), is currently used as well because of its similarity to the composition of bone and its biocompatibility and bioactivity which stimulates in vivo bone growth. However, hydroxyapatite cannot handle heavy loads of pressure, in which scientists are creating composites out of HA and natural hydrogels made of collagen, chitosan, and other polymers.
The main challenge when constructing bones is to engineer ones that have well-constructed vascular systems which is essential for blood flow that would help rebuild damaged bone tissues.
SKIN
The skin is the largest organ of the human body and is essential for survival as it acts as the barrier between the environment and microorganism, regulates body temperature, and retains water.
Skin is only organ which is constantly growing and regenerating as a result of the presence of keratinocytes, or epidermal stem cells, in the epidermis. However, when a large percent of skin is lost from severe burns, for example, the skin does not fully recover to its previous state: hair follicles cannot grow back and the skin aesthetic is ruined.
There are several therapies for damaged skin. One common therapy is applying a skin graft over the damaged area. Autologous cells are used to make grafts which covers wound sites and promotes healing and reintegration with the skin. However, those who do not have much skin left must resort to skin allografts or xenografts, which is risky due to immunological rejection and immunosuppreseant drugs. Often times, these alternatives have been used as temporary therapies for patients.
Skin tissue engineering seeks to improve current graft techniques by setting up a biodegradable scaffold, in this case, a matrix, which a large amount of cells that can proliferate and be applied to the skin at once instead of layering autografts multiple times which is costly and time-consuming. Traditional autograft methods also do not promise quick healing and the restoration of skin aesthetic. Current in vivo treatments still require the use of autografts. Integra®, for example, allows for preliminary dermal regeneration, quicker healing, and better appearances, but still needs and autograft to complete treatment reepithelialize the wound. In vitro products are not widely used because of inefficacy and impracticality.
Skin TE treatments and technologies have been available for 30 years now, and continues to develop and improve. Scientists now face challenges such as reestablish hair follicles and sweat glands. Also, induced plurpotent stem cells provide more patient-specific therapies which lead to a large number of cell sources and decreases the risk of immunological rejection, which no one want to go through.

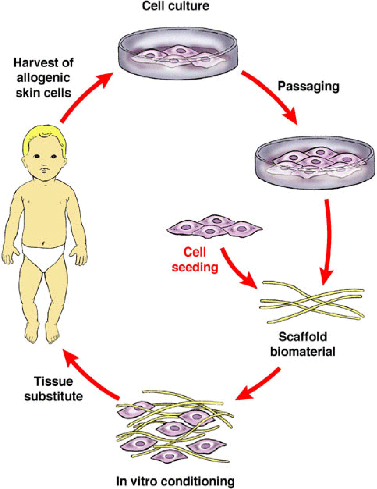
Anatomy of the skin (I7.14)
Cycle of skin tissue engineering treatment (I7.15)